Regional conditions shape the food–energy–land nexus of low-carbon indoor farming - Nature.com
Abstract
Modern greenhouses and vertical farming projects promise increased food output per unit area relative to open-field farming. However, their high energy consumption calls for a low-carbon power supply such as solar photovoltaic and wind, which adds to cost and overall land footprint. Here we use geospatial and mathematical modelling to compare open-field and two indoor farming methods for vegetable production in nine city-regions chosen globally with varying land availability, climatic conditions and population density. We find that renewable electricity supply is more costly for greenhouses per unit energy demand satisfied, which is due to the greater fluctuation in their energy demand profile. However, greenhouses have a lower energy demand per unit food output, which makes them the least land-intensive option in most of the analysed regions. Our results challenge the land-savings claims of vertical farming compared with open-field production. We also show that regionalizing vegetable supply is feasible in most regions and give recommendations based on the regional context.
This is a preview of subscription content
Access options
Subscribe to Journal
Get full journal access for 1 year
119,01 €
only 9,92 € per issue
Tax calculation will be finalised during checkout.
Buy article
Get time limited or full article access on ReadCube.
$32.00
All prices are NET prices.
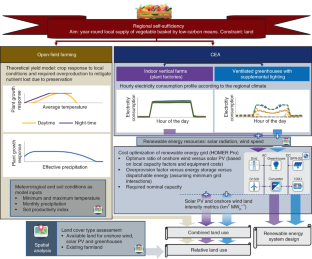
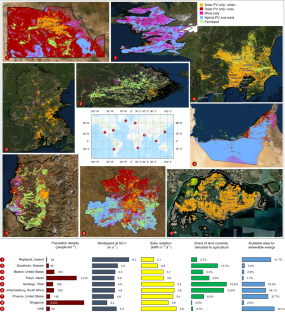
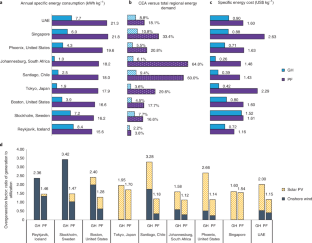
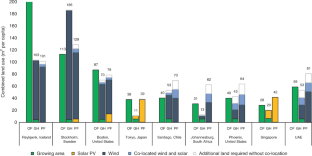
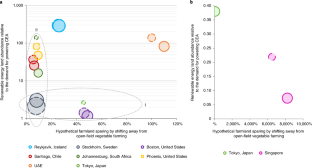
Data availability
The majority of the data that are important to interpret, verify and/or extend this work have been included either directly in the main text or detailed in Supplementary Tables 2 and 3. Further primary data are available at https://doi.org/10.6084/m9.figshare.14778804.v1 (ref. 95) and include the energy demand curves, vegetable basket and yield factor data, and the output from HOMER Pro and ArcGIS Pro. Publicly available third-party datasets are described in the Methods and are listed in refs. 58,59,60,61,62,64. Source data are provided with this paper.
Code availability
All computations are fully described in the Methods and in the Supplementary Information. HOMER Pro files and Excel files to generate the figures can be obtained from the corresponding author on reasonable request.
References
Béné, C. Resilience of local food systems and links to food security—a review of some important concepts in the context of COVID-19 and other shocks. Food Secur. 12, 805–822 (2020).
Damage and Losses from Climate-Related Disasters in Agricultural Sectors (FAO, 2015); www.fao.org/3/a-i5128e.pdf
Vogel, E. et al. The effects of climate extremes on global agricultural yields. Environ. Res. Lett. 14, 054010 (2019).
Davis, K. F., Downs, S. & Gephart, J. A. Towards food supply chain resilience to environmental shocks. Nat. Food 2, 54–65 (2021).
Shoup, M. E. Pandemic accelerates demand for fresh food and gives rise to the 'contemporary consumer' (2020); https://www.foodnavigator-usa.com/Article/2020/10/28/Pandemic-accelerates-demand-for-fresh-food-and-gives-rise-to-the-contemporary-consumer
Richards, T. J. & Rickard, B. COVID-19 impact on fruit and vegetable markets. Can. J. Agric. Econ. 68, 189–194 (2020).
Zasada, I. et al. Food beyond the city-analysing foodsheds and self-sufficiency for different food system scenarios in European metropolitan regions. City Cult. Soc. 16, 25–35 (2019).
Growing Beyond the Hype: Controlled Environment Agriculture (S2G Ventures, 2020).
2020 Global CEA Census Report (Agritecture, 2020).
Benke, K. & Tomkins, B. Future food-production systems: vertical farming and controlled-environment agriculture. Sustain. Sci. Pract. Policy 13, 13–26 (2017).
Barbosa, G. L. et al. Comparison of land, water, and energy requirements of lettuce grown using hydroponic vs conventional agricultural methods. Int. J. Environ. Res. Public Health 12, 6879–6891 (2015).
Graamans, L., Baeza, E., van den Dobbelsteen, A., Tsafaras, I. & Stanghellini, C. Plant factories versus greenhouses: comparison of resource use efficiency. Agric. Syst. 160, 31–43 (2018).
Avgoustaki, D. D. & Xydis, G. Indoor vertical farming in the urban nexus context: business growth and resource savings. Sustain. 12, 1–18 (2020).
Teo, Y. L. & Go, Y. I. Techno-economic-environmental analysis of solar/hybrid/storage for vertical farming system: a case study. Malaysia. Renew. Energy Focus 37, 50–67 (2021).
Herrero, M. et al. Innovation can accelerate the transition towards a sustainable food system. Nat. Food 1, 266–272 (2020).
World Energy Outlook 2020 (IEA, 2020); https://www.iea.org/reports/world-energy-outlook-2020
Saunders, P. J. Land Use Requirements of Solar and Wind Power Generation: Understanding a Decade of Academic Research (Energy Innovation Reform Project, 2020).
Gao, L. & Bryan, B. A. Finding pathways to national-scale land-sector sustainability. Nature 544, 217–222 (2017).
Xie, Z. et al. Conservation opportunities on uncontested lands. Nat. Sustain. 3, 9–15 (2020).
Foley, J. A. et al. Global consequences of land use. Science 309, 570–574 (2005).
Graham-Rowe, D. Agriculture: Beyond food versus fuel. Nature 474, S6–S8 (2011).
Calvert, K. & Mabee, W. More solar farms or more bioenergy crops? Mapping and assessing potential land-use conflicts among renewable energy technologies in eastern Ontario, Canada. Appl. Geogr. 56, 209–221 (2015).
Nie, Y. et al. A food–energy–water nexus approach for land use optimization. Sci. Total Environ. 659, 7–19 (2018).
Leung Pah Hang, M. Y., Martinez-Hernandez, E., Leach, M. & Yang, A. Insight-based approach for the design of integrated local food–energy–water systems. Environ. Sci. Technol. 51, 8643–8653 (2017).
Namany, S., Al-Ansari, T. & Govindan, R. Optimisation of the energy, water, and food nexus for food security scenarios. Comput. Chem. Eng. 129, 106513 (2019).
Sasse, J.-P. & Trutnevyte, E. Regional impacts of electricity system transition in Central Europe until 2035. Nat. Commun. 11, 4972 (2020).
Poggi, F., Firmino, A. & Amado, M. Planning renewable energy in rural areas: impacts on occupation and land use. Energy 155, 630–640 (2018).
Sliz-Szkliniarz, B. Assessment of the renewable energy-mix and land use trade-off at a regional level: a case study for the Kujawsko-Pomorskie Voivodship. Land Use Policy 35, 257–270 (2013).
The Water–Energy–Food Nexus: A New Approach in Support of Food Security and Sustainable Agriculture (FAO, 2014); http://www.fao.org/3/bl496e/bl496e.pdf
De Ruiter, H., Macdiarmid, J. I., Matthews, R. B. & Smith, P. in Land Use Competition. Human–Environment Interactions Vol. 6 (eds Niewöhner J. et al.) 247–261 (Springer, 2016); https://doi.org/10.1007/978-3-319-33628-2
Mead, B. R. et al. Is urban growing of fruit and vegetables associated with better diet quality and what mediates this relationship? Evidence from a cross-sectional survey. Appetite 163, 105218 (2021).
Kinnunen, P. et al. Local food crop production can fulfil demand for less than one-third of the population. Nat. Food 1, 229–237 (2020).
Peters, C. J., Bills, N. L., Lembo, A. J., Wilkins, J. L. & Fick, G. W. Mapping potential foodsheds in New York State by food group: an approach for prioritizing which foods to grow locally. Renew. Agric. Food Syst. 27, 125–137 (2012).
Martins-Turner, K., Grahle, A., Nagel, K. & Dietmar, G. Electrification of urban freight transport—a case study of the food retailing industry. Procedia Comput. Sci. 170, 757–763 (2020).
Hemming, S., De Zwart, F., Elings, A., Righini, I. & Petropoulou, A. Remote control of greenhouse vegetable production with artificial intelligence—greenhouse climate, irrigation, and crop production. Sensors (Basel) 19, 1807 (2019).
Drew, F. & Rhee, K. S. Energy use, cost, and product quality in preserving vegetables at home by canning, freezing, and dehydration. J. Food Sci. 45, 1561–1565 (1980).
Li, M., Ho, K. K. H. Y., Hayes, M. & Ferruzzi, M. G. The roles of food processing in translation of dietary guidance for whole grains, fruits, and vegetables. Annu. Rev. Food Sci. Technol. 10, 569–596 (2019).
Rae, C. & Bradley, F. Energy autonomy in sustainable communities—a review of key issues. Renew. Sustain. Energy Rev. 16, 6497–6506 (2012).
Laugs, G. A. H., Benders, R. M. J. & Moll, H. C. Balancing responsibilities: effects of growth of variable renewable energy, storage, and undue grid interaction. Energy Policy 139, 111203 (2020).
Kazem, H. A., Al-Badi, H. A. S., Al Busaidi, A. S. & Chaichan, M. T. Optimum design and evaluation of hybrid solar/wind/diesel power system for Masirah Island. Environ. Dev. Sustain. 19, 1761–1778 (2017).
Khattab, N. M. et al. Hybrid renewable energy system for water desalination: a case study for small green house hydroponic cultivation in Egypt. ARPN J. Eng. Appl. Sci. 11, 12380–12390 (2016).
Johlas, H., Witherby, S. & Doyle, J. R. Storage requirements for high grid penetration of wind and solar power for the MISO region of North America: a case study. Renew. Energy 146, 1315–1324 (2020).
Perez, M., Perez, R., Rábago, K. R. & Putnam, M. Overbuilding & curtailment: the cost-effective enablers of firm PV generation. Sol. Energy 180, 412–422 (2019).
Dujardin, J., Kahl, A., Kruyt, B., Bartlett, S. & Lehning, M. Interplay between photovoltaic, wind energy and storage hydropower in a fully renewable Switzerland. Energy 135, 513–525 (2017).
Weidner, T., Yang, A. & Hamm, M. W. Energy optimisation of plant factories and greenhouses for different climatic conditions. Energy Convers. Manag. 243, 114336 (2021).
Miskin, C. K. et al. Sustainable co-production of food and solar power to relax land-use constraints. Nat. Sustain. 2, 972–980 (2019).
New Energy Outlook 2019 (Bloomberg, 2019).
Iocola, I. et al. Sustainability assessment of organic vegetable production using a qualitative multi-attribute model. Sustain. 10, 3820 (2018).
Farm and Building in Circular Symbiosis (RISE, 2020); https://www.ri.se/en/our-stories/farm-and-building-in-circular-symbiosis
FAOSTAT Statistics Database (FAO, 2021); http://www.fao.org/faostat/en/#home
EU Fruit and Vegetables Market Observatory. The Tomato Market in the EU, Vol. 1: Production, Areas and Yields (European Commission, 2020); https://ec.europa.eu/info/sites/info/files/food-farming-fisheries/farming/documents/tomatoes-production_en.pdf
Willett, W. et al. Food in the Anthropocene: the EAT–Lancet Commission on healthy diets from sustainable food systems. Lancet 393, 447–492 (2019).
Sokolow, J., Kennedy, G. & Attwood, S. Managing crop tradeoffs: a methodology for comparing the water footprint and nutrient density of crops for food system sustainability. J. Clean. Prod. 225, 913–927 (2019).
Greater Tokyo Area (Wikipedia, accessed 25 February 2021); https://en.wikipedia.org/wiki/Greater_Tokyo_Area
Greater Boston Metropolitan region (Wikipedia, accessed 25 February 2021); https://en.wikipedia.org/wiki/Greater_Boston
Mälaren Valley (Wikipedia, accessed 25 February 2021); https://en.wikipedia.org/wiki/Malaren_Valley
GADM database of Global Administrative Areas, version 3.6 (GADM, accessed 25 February 2021); https://gadm.org/download_world.html
Gridded Population of the World v.4 (GPWv4): Population Count Adjusted to Match 2015 Revision of UN WPP Country Totals, Revision 11 (CIESIN, 2018).
Fick, S. E. & Hijmans, R. J. WorldClim 2: new 1-km spatial resolution climate surfaces for global land areas. Int. J. Climatol. 37, 4302–4315 (2017).
Buchhorn, M. et al. Copernicus Global Land Service: Land Cover 100m: collection 3: epoch 2019: Globe v.3.0.1 https://doi.org/10.5281/zenodo.3939050 (2020).
CORINE Land Cover (European Environment Agency, 2018); https://land.copernicus.eu/pan-european/corine-land-cover/clc2018
GAP/LANDFIRE National Terrestrial Ecosystems 2011 (US Geological Survey, 2016); https://doi.org/10.5066/F7ZS2TM0
Food Farms in Singapore (Singapore Food Agency, accessed 16 March 2021); https://www.sfa.gov.sg/food-farming/food-farms/farming-in-singapore
Environmental Data Explorer—The Environmental Database (United Nations Environment Programme, accessed 10 March 2021); http://geodata.grid.unep.ch/results.php
Willmott, C. & Feddema, J. A more rational climatic moisture index. Prof. Geogr. 44, 84–88 (1992).
Ramankutty, N., Foley, J. A., Norman, J. & McSweeney, K. The global distribution of cultivable lands: current patterns and sensitivity to possible climate change. Glob. Ecol. Biogeogr. 11, 377–392 (2002).
Nadal, A. et al. Urban planning and agriculture. Methodology for assessing rooftop greenhouse potential of non-residential areas using airborne sensors. Sci. Total Environ. 601–602, 493–507 (2017).
McDonald, R. I., Fargione, J., Kiesecker, J., Miller, W. M. & Powell, J. Energy sprawl or energy efficiency: climate policy impacts on natura...
Comments
Post a Comment